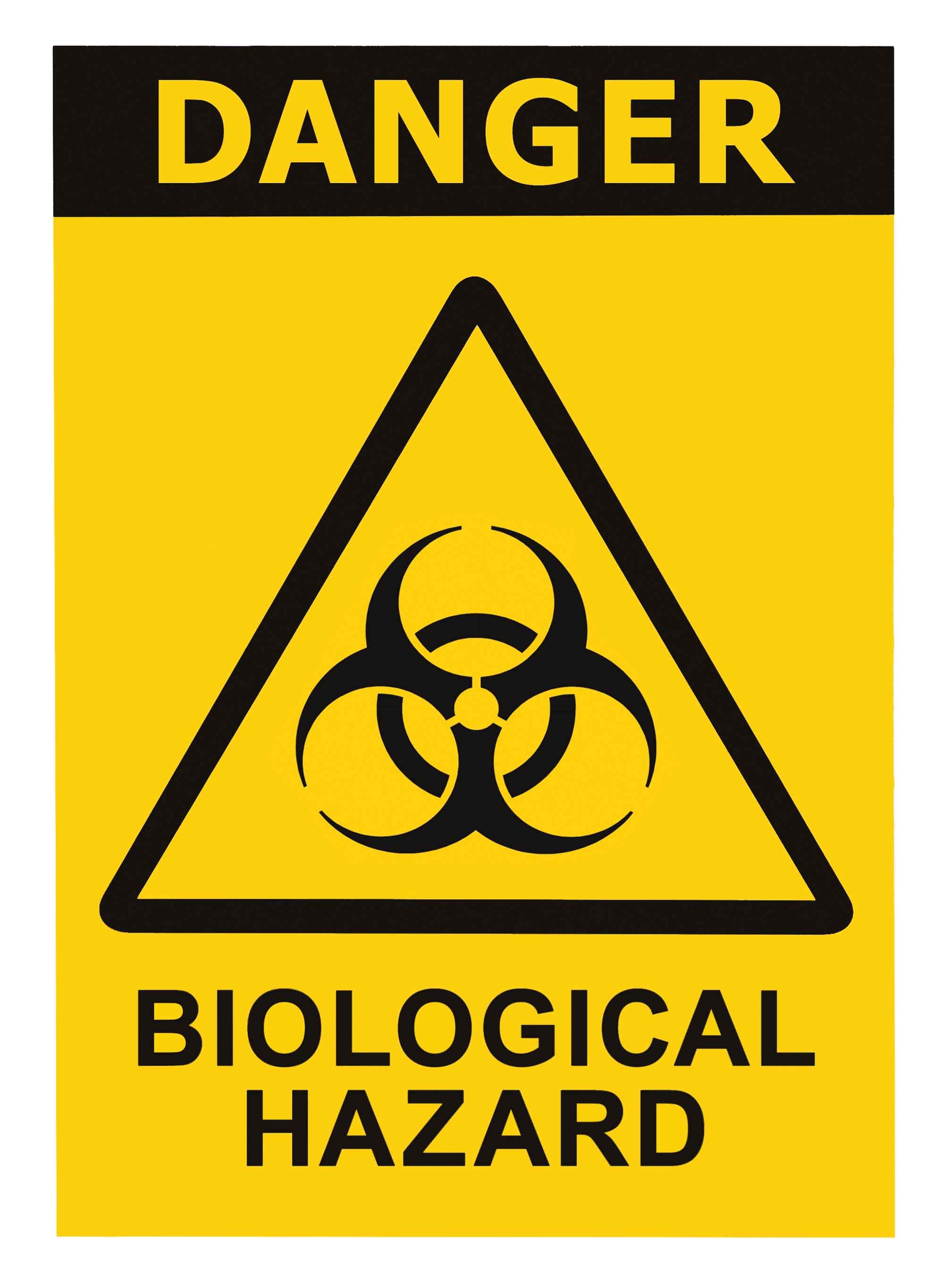
Chlorine is used in water treatment at the city, small-systems, and even homeowner level. In fact, I believe that water chlorination is one of the single greatest contributions to increasing human longevity through significantly reducing the proliferation of waterborne diseases.
One hundred and fifty years ago, much of the USA’s water supply was teeming with various forms of aquatic organisms. Waterborne diseases, such as cholera, typhoid, and dysentery, were a serious health problem, and they are still major concerns in third-world nations where over a billion people lack clean drinking water and almost two billion lack adequate sewage systems.
In 1992, the World Bank rated drinking water as first on its list of preventable environmental hazards worldwide. Since 1991 the largest cholera epidemic in recent history infected over 800,000 people from Peru to Mexico.
Waterborne microorganisms include coliforms and heterotrophic bacteria, viruses, and protozoa. These organisms range in size from extremely small viruses to relatively large cysts. They also vary greatly in the nature of their structure, lifecycle, and reproduction characteristics. Pathogenic microorganisms occur naturally in lakes, streams, reservoirs, and most surface water sources. Groundwater supplies are now becoming a subject of increasing concern, because enteric viruses and other organisms can leach into the groundwater system from the land application or burial of sewage sludge and other treatment wastes.
Since water utilities first began using filtration and disinfection systems a century ago, the risk of disease from drinking water in first-world countries has been greatly reduced. Despite the significant progress that has been made, there are still numerous disease cases resulting from contaminated drinking water in the United States. Health risks from aquatic pathogens range from mild gastrointestinal distress to systemic disease and, in severe cases, even death.
There are a number of reasons to chlorinate, such as:
- Control of certain nuisance bacteria
- Control of certain pathogenic bacteria
- Oxidation of Arsenic
- Oxidation of Iron
- Oxidation of Manganese
- Oxidation or Hydrogen Sulfide
- Oxidation of organic materials that can cause tastes, odors, or color in water
The water that most of us drink is stored, treated and distributed to our homes by public and private water utilities.
Algae, bacteria, fungi and viruses can often be found in untreated water. Americans have grown to expect a safe drinking water supply but achieving that level of safety is a complex task.
The Benefits of Municipal Chlorination
There are nearly 250,000 public water supply systems in the United States, serving everything from the smallest towns to major metropolitan centers. Ninety percent of the population receives its water through these community water systems, with the rest using private wells or other individual sources. Chlorine and its compounds are currently used by over 98 percent of all U.S. water utilities that disinfect drinking water; It is a cheap and efficient killer.
By adding chlorine and its compounds to drinking water, almost all organisms living in the water are killed. Chlorine remains in the water as it is distributed to homes and businesses, thereby retaining much of its ability to continue killing.
Although chlorine’s disinfectant value has been known for nearly a century, the mechanism by which the compound kills or inactivates microorganisms is still not completely understood.
Chlorination can deactivate microorganisms by a variety of mechanisms, such as damage to cell membranes, inhibition of specific enzymes, destruction of nucleic acids, and mechanisms. The effectiveness of the chlorination process depends upon a variety of factors, including chlorine concentration and contact time, water temperature, pH value, and level of turbidity.
Chlorination is the cheapest, most effective way to disinfect water that is stored, processed and distributed to homes and businesses at a municipal level. It is a cheap, efficient killer that helps protect us all from deadly microbial diseases.
The Risks of Chlorination
When chlorine is exposed to organic contaminants, certain disinfection byproducts (DBP’s) are formed. For example, naturally occurring fulvic and humic acids in water will react with chlorine to form a toxic soup containing numerous compounds such as trihalomethanes, haloacetic acids, trichloroacetic acid, and others. Chlorine will also react with the biofilm of heterotrophic bacteria so common in piping systems and many water treatment devices. Over 600 disinfection byproducts have been identified in drinking water treated by chlorine or chloramine.
Epidemiological studies have related exposure to chlorine disinfection byproducts with birth defects, pregnancy complications, certain cancers like bladder, rectal and kidney (recent studies suggest there might even be a causal relationship between chlorine byproducts and breast cancer), respiratory stress, eye irritation, skin damage, headaches and fatigue.
Traditionally, the risk of chlorine and disinfection byproducts has been downplayed, since the risk of non-chlorination is significantly greater. In fact the World Health Organization (WHO) recently stated – “the risk of death from pathogens is at least 100 to 1000 times greater than the risk of cancer from disinfection by-products (DBPs) {and} the risk of illness from pathogens is at least 10,000 to 1 million times greater than the risk of cancer from DBPs” Essentially, the consumer is being told that they must choose between illness and/or death from disease and microorganisms, or a steady decline in quality of life from the permanent damage caused by chlorine and its byproducts of disinfection.
It is also important to understand what chlorine does in water at different temperatures and pH levels. Chlorine is soluble in water to about 7,000 ppm at 68°F. It reacts with water forming hypochlorous acid (HOCl). In alkali solutions hypochlorous acid dissociates forming hypochlorite (OCl-). Chlorine, hypochlorous acid and hypochlorite exist together in equilibrium.
Cl2+ H2O
HOCl
OCl
-Increasing pH →
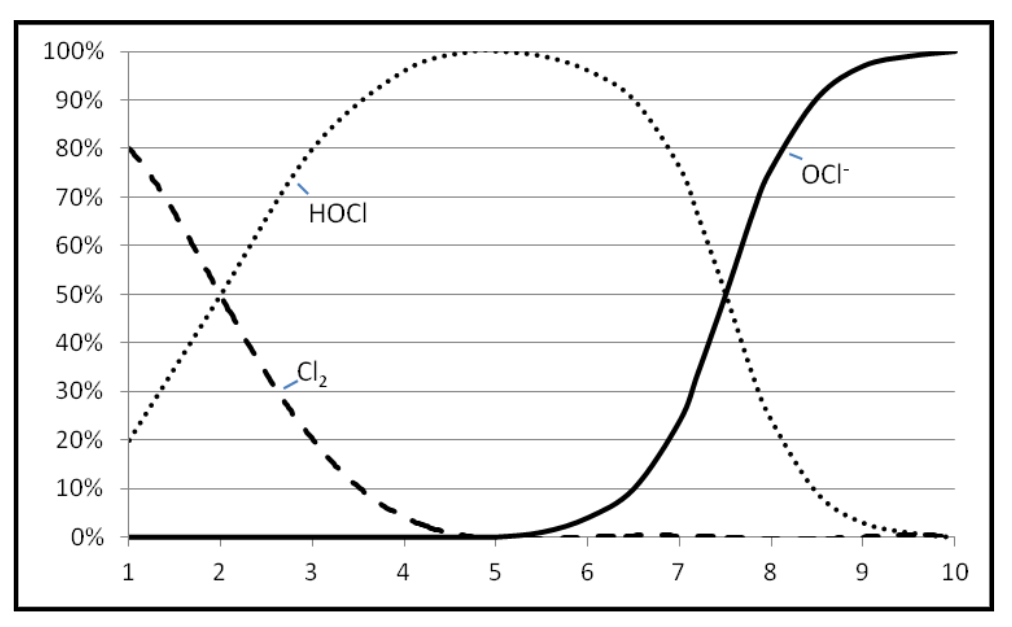
Bleach decomposes when heated, forming sodium chlorate and sodium chloride:
3 NaClO → NaClO3 + 2 NaCl
When mixed with water, it slowly decomposes, releasing sodium &, chloride ions along with hydroxyl radicals:
NaClO + H2O → Na+ + Cl− + 2 HO• The sodium and chloride will eventually create Sodium Chloride salt.
Sodium Chloride will contribute to corrosion, so care should be taken when using it on a continuous basis.
Adding Chlorine to Water
One of the easiest ways to chlorinate at the residential level is with Sodium Hypochlorite bleach.
Sodium hypochlorite’s chemical formula is NaClO. It is composed of a sodium cation (Na+) and a hypochlorite anion (ClO−). It can also be described as the sodium salt of hypochlorous acid.
It important to understand that Sodium hypochlorite is practically and chemically distinct from chlorine itself. Bleach is commonly used as a biocide to control slime and bacterial growth in water systems, as well as to address iron, manganese, hydrogen sulfide in water. Interestingly enough, you can develop a mild bleach by reacting ozone with a brine solution; the broad reaction is NaCl + O3 → NaClO + O2.
Remember that when adding chlorine to a drinking water supply that it must be certified to meet NSF/ANSI 60: Drinking Water Chemicals Health Effects
Important Safety Warning
Sodium hypochlorite is a strong oxidizer.
Oxidation reactions are corrosive.
Bleach can burn the skin and cause eye damage, especially at higher concentrations.
Using household bleach to shock treat a tank
Typical household bleach contains approximately 5.25% of free reactive chlorine.
When shock treating a water system, it is critical to use enough chlorine to reach an available chlorine level of at least 50 ppm (parts per million). Remember that 1 ppm = 1 milligram/Liter.
If you use too little bleach, you may not eliminate the bacteriological contaminants.
In order to determine how much bleach to add you must know the actual volume water being disinfected.
For example, if you’re working with 1,000 gallon water storage tank, here is how you would determine how much bleach to add to disinfect the full tank:
->Multiply 1,000-gal x 50 ppm – 1,000 x (50/1,000,000) = 0.05 gal of chlorine
This is the amount of pure chlorine you would need to add to mix to 50 ppm.
->Since bleach is only 5.25% chlorine or in other words 1 gallon of bleach = 0.0525 gallons of chlorine.
So, in this case 0.05 gal/0.0525 = 0.9524 gallons of bleach. Rounding up to the nearest gallon would mean that ~1 Gallon of bleach would be used for the 1,000 Gallon tank.
Determining Chlorine Demand
Expressed simply:
Chlorine Demand = Chlorine added concentration (mg/L) – Chlorine residual measured concentration (mg/L)
It is important to understand the chlorine demand of the water before attempting chlorination because your dosage and contact times are dependent on this demand.
Materials Needed:
- 100mL Water sample.
- 1% Chlorine solution – Sodium Hypochlorite bleach is the easiest
- Graduated cylinders or pipettes for measuring volumes.
- Stirring rod or magnetic stirrer for mixing (non-metallic).
- Free chlorine test kit: Such as DPD (N,N-diethyl-p-phenylenediamine) test cube, color wheel, digital colorimeter, or decent chlorine test strips. OTO (Orthotolidine) should not be used since it can’t differentiate between free and total chlorine. If you find that you’re looking at Cl2 levels higher than 10 mg/L, use a FAS-DPD (ferrous ammonium sulfate) test instead of just DPD.
- Glass or lab-grade plastic Beakers for holding samples and mixing.
- Timer: To measure contact time.
Procedure:
- Prepare the Chlorine Solution:
- Prepare a standard chlorine solution of known concentration. For instance, you can use a 1% sodium hypochlorite solution.
- Measure the Initial Chlorine Concentration:
- Take an initial measurement of the chlorine concentration of your stock solution using the chlorine test kit to ensure accuracy.
- Add Chlorine to Water Sample:
- Measure a known volume of the water sample (e.g., 100 mL) into a clean beaker.
- Add a measured volume of the chlorine solution to the water sample. For a starting point, you can add 1 mL of the chlorine solution.
- Mix and Allow Contact Time:
- Mix the water sample thoroughly using a stirring rod or magnetic stirrer (no metallic stirrers).
- Allow the mixture to sit for a predetermined contact time, typically 30 minutes. This ensures the chlorine has time to react with the constituents in the water.
- Measure Residual Chlorine:
- After the contact time, measure the residual chlorine concentration in the water sample using the DPD test kit or colorimeter.
- Calculate Chlorine Demand:
- Chlorine demand is calculated as the difference between the initial chlorine concentration added and the residual chlorine concentration after the contact time.
Example Calculation:
- Initial Chlorine Solution Concentration: 100 mg/L
- Volume of Chlorine Solution Added: 1 mL to 100 mL of water sample
- This gives an initial chlorine concentration in the water sample of 1.0 mg/L
- Residual Chlorine Concentration After 30 Minutes: 0.2 mg/L
- Chlorine Demand: 1.0 mg/L – 0.2 mg/L, which is 0.8 mg/L
Notes:
- Plan on running multiple tests with different chlorine doses to determine the optimal chlorine demand.
- If chlorine demand is inordinately high, investigate why and bench-test pretreatment for organic removal before chlorination.
- You might need to experiment with pH stabilization – consult with your local MWS.
- Handle all chemicals, especially chlorine, with appropriate safety precautions, including gloves, goggles, and working in a well-ventilated area
Quick field-test method:
- Making a 1% Bleach Solution:
- Pour 1 teaspoon of bleach into a measuring jug that can hold at least 2 cups.
- Add water to the jug until it reaches 1 cup (240 mL).
- Stir well with a non-metallic stirrer
- You now have a 1% bleach solution.
- Prepare Drops of the 1% Bleach Solution:
- Use a dropper to draw up the 1% bleach solution.
- Mix the Bleach Solution with the Water Sample:
- Take 1 cup of your water sample and pour it into a clean container that can hold at least 2 cups.
- Add 10 drops of the 1% bleach solution to the water sample.
- Stir well and let it sit for 30 minutes.
- Test for Chlorine:
- After 30 minutes, test for free chlorine with strips or DPD
- If the test strip shows little or no chlorine, your water has a high chlorine demand.
- If the test strip shows a lot of chlorine, your water has a low chlorine demand.
Example:
- Start: 1 cup of water, add 10 drops of 1% bleach solution.
- Wait: Stir and let it sit for 30 minutes.
- Test: Dip the test strip and compare the color.
If you added 10 drops of bleach solution and there’s no chlorine left after 30 minutes, your water needed all that chlorine to react with stuff in it. If there’s still some chlorine, your water needed less chlorine.
* * *
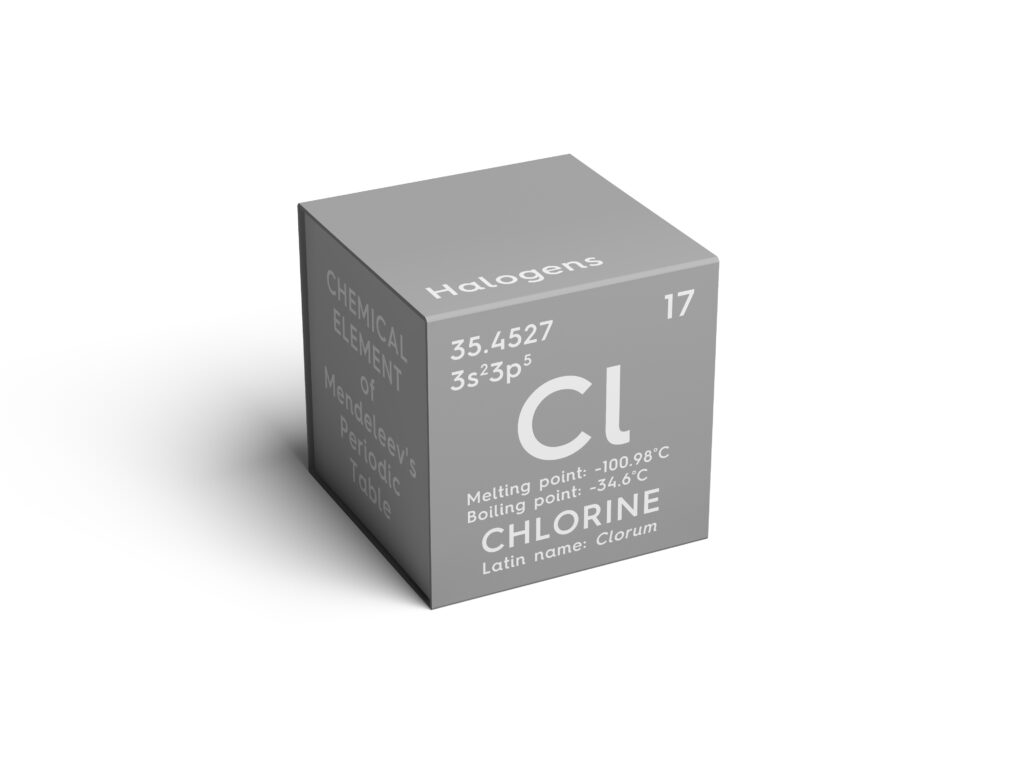
DEFINITIONS
Trihalomethanes – Chemical Compounds where three of the four hydrogen atoms of methane are replaced by halogen atoms. Many trihalomethanes are used in industry and the home as solvents or refrigerants. THM’s are generally considered environmental pollutants, and many are actually carcinogenic. The USEPA currently limits THM’s (chloroform, bromoform, bromodichloromethane, and dibromochloromethane) to 80 ppb in treated water,
Haloacetic Acids – Carboxylic acids where a halogen atom replaces a hydrogen atom in acetic acid. Haloacetic acids in varying forms are common disinfection byproducts of chlorination.
Chloroform – A trihalomethane reagent/solvent, considered an environmental hazard. Chloroform is often inadvertently synthesized during the water treatment process when chlorine and related compounds are added to water. The US Department of Health and Human Resources National Toxicology Program’s eleventh report on carcinogens implicates chloroform as a human carcinogen; a designation equivalent to International Agency for Research on Cancer class 2A. It has been most readily associated with hepatocellular cancer. Chloroform once appeared as an ingredient in toothpastes, cough syrups, ointments, and other pharmaceuticals, and was banned in the USA as a consumer product ingredient in 1976.